
shredded4summer
Pretty Boy Chad
- Joined
- Jul 8, 2023
- Posts
- 4,169
- Reputation
- 6,882
Table of Contents
- Introduction
1.1 Overview of Height Augmentation Research
1.2 Rationale for Terrestrial Decompression Therapy - Theoretical Foundations
2.1 Astronaut Height Gain in Microgravity
2.2 Biomechanical Principles of Axial Elongation
2.3 Viscoelastic and Plastic Tissue Adaptation - Equipment and Apparatus
3.1 Cranial Traction Harness
3.2 Ankle Cuff Traction System
3.3 Femoral and Tibial Extension Modules
3.4 Nocturnal Passive Traction Unit (NTU) - Decompression Protocols
4.1 Hourly Cyclic Decompression Stimulus (HCDS)
4.2 Nocturnal Passive Elongation Protocol (NPEP)
4.3 Appendicular Extension: Femur and Tibia
4.4 Force Parameters and Calibration - Mechanobiological Mechanisms
5.1 Intervertebral Disc Expansion
5.2 Cortical and Periosteal Response
5.3 Spinal and Postural Recalibration - Safety Considerations and Risks
6.1 Physiological Stress Thresholds
6.2 Contraindications and Adverse Effects
6.3 Monitoring and Adjustment Protocols - Expected Outcomes
7.1 Projected Height Gains
7.2 Structural and Postural Impacts
7.3 Limitations and Reversibility - Conclusion and Future Research Directions
- Hormonal Assistance (pubertycels only)
- References and Supporting Literature
Theoretical Framework: Spinal Elongation in Microgravity
NASA-documented data indicates that astronauts may experience vertical elongation of up to 5.7 cm (2.25 inches) during prolonged exposure to microgravity. The mechanism is spinal decompression due to reduction of axial gravitational loading, permitting expansion of intervertebral disc spaces.
This phenomenon is temporary in space due to reloading upon reentry. However, with persistent and repetitive axial unloading stimuli, we hypothesize a more permanent adaptation through plastic deformation of soft tissues and postural recalibration.
Proposed Modality: Longitudinal Skeletal Decompression Therapy (LSDT)
Objective:
To induce measurable height gains (2–3 inches) through mechanical traction protocols mimicking the zero-G environment’s effect on the axial and appendicular skeleton.
Mechanism:
- Tensile stress induces elongation of intervertebral discs, femoral shaft, and tibial diaphysis.
- Sustained traction stimulates fibrocartilaginous and periosteal plasticity.
- Sleep-based traction maintains post-decompression expansion.
Equipment Specifications
- Cranial Traction Harness: Anchored to a calibrated motorized winch system.
- Bilateral Ankle Cuffs: Connected to counteracting downward traction arms.
- Femoral & Tibial Modular Harness System: Custom fit for mid-diaphyseal application.
- Nighttime Traction Unit (NTU): Low-load sustained tension module for passive elongation.
Daytime Protocol: Hourly Cyclic Decompression Stimulus (HCDS)
Duration: 5 minutes per hour, 18 cycles per day
Total Active Decompression Time: 1.5 hours/day
Traction Vectoring:
- Cranial upward vector: 25–30% of bodyweight
- Pedal downward vector: Equal and opposite force
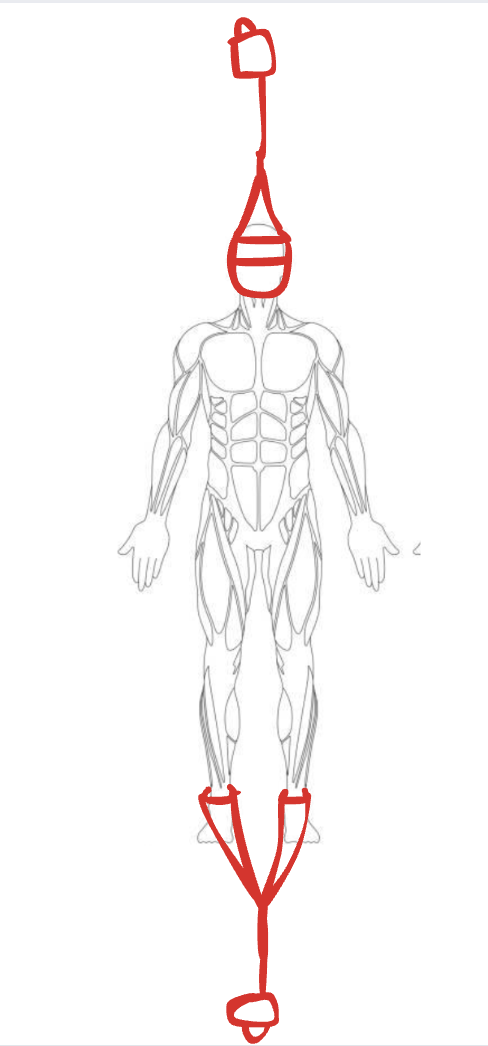
Biomechanical Target:
- Expansion of lumbar and thoracic intervertebral discs
- Postural realignment via axial recalibration
- Stimulation of vertebral ligamentous plasticity
Nocturnal Passive Elongation Protocol (NPEP)
Duration: 10 hours (overnight)
Traction Load: 10–15% bodyweight (static)
Focus: Maintenance of daytime elongation through passive soft tissue compliance during somnolence.
Mechanism: Facilitates long-term tissue adaptation via diurnal cyclical stress-relief loading.
Appendicular Skeletal Extension Modules
Femoral Traction Protocol (FTP)
- Traction Site: Greater trochanter to supracondylar ridge
- Direction: Axial elongation
- Duration: 5 min/hour, alternating with tibial set
- Goal: Micro-tensile adaptation of cortical bone and periosteum
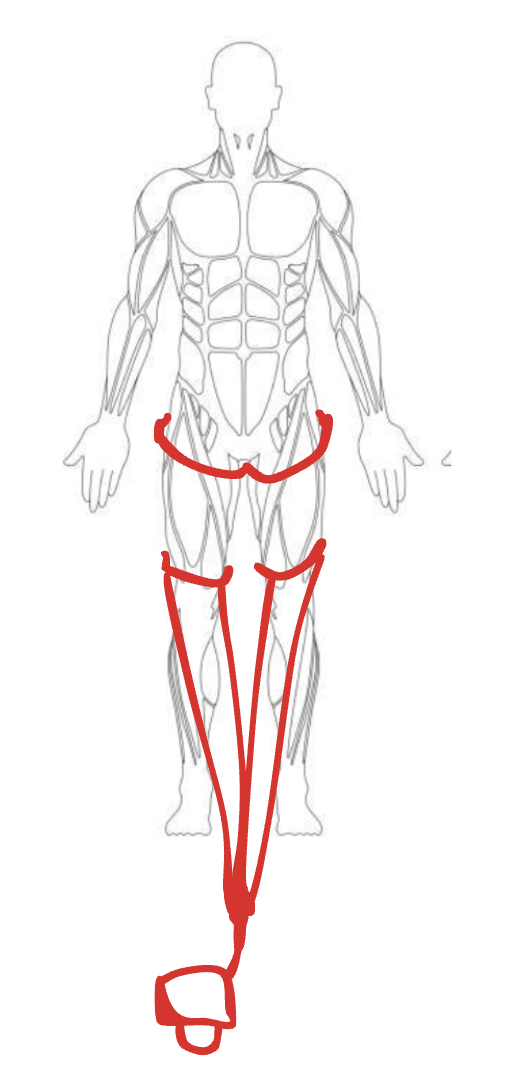
Tibial Traction Protocol (TTP)
- Traction Site: Distal patella to talocrural junction
- Force Parameters: 20–25% bodyweight in a downward vector
- Objective: Encourage tibial axis lengthening via induced mechanical creep
Both routines are alternated in 60-minute cycles during waking hours.
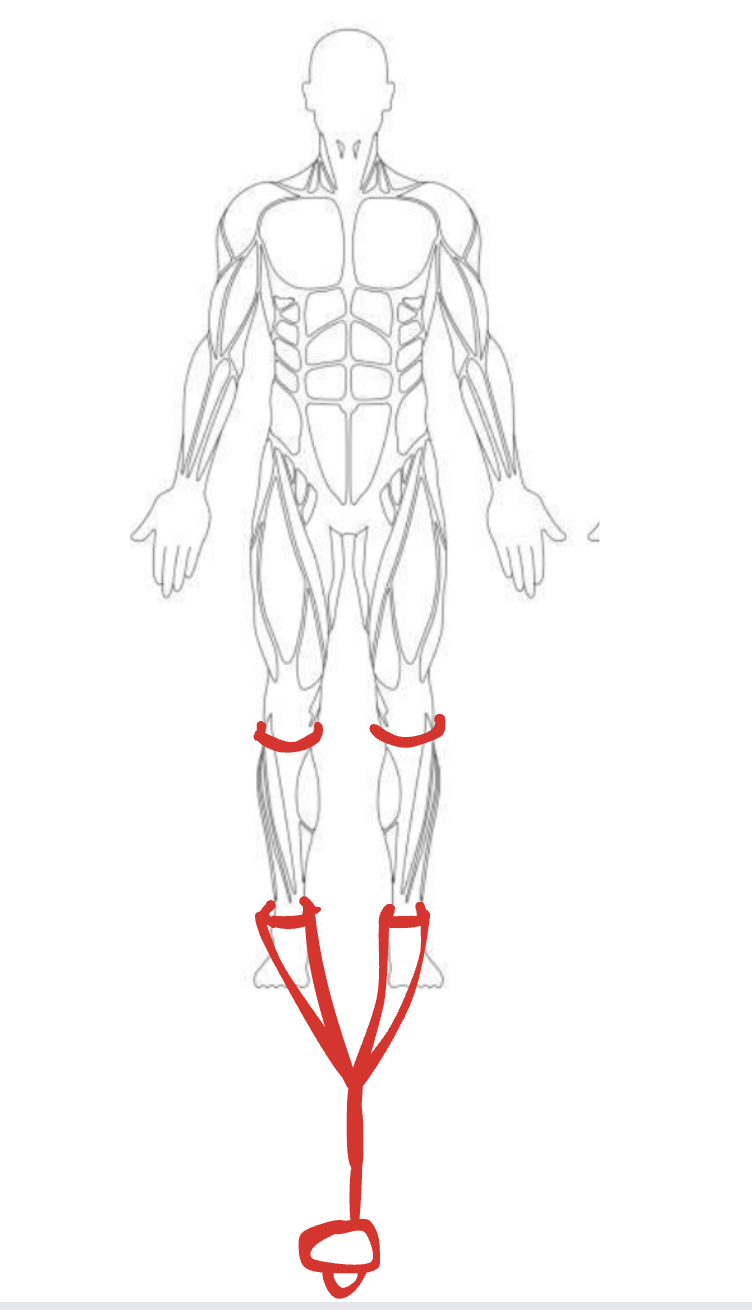
Safety and Adaptation Considerations
While not clinically approved, LSDT is theorized to promote:
- Viscoelastic tissue elongation
- Postural height normalization
- Potential bone remodeling under Wolff’s Law (if performed long-term)
Conclusion
Through rigorous implementation of the Longitudinal Skeletal Decompression Therapy protocol, individuals may feasibly attain stature augmentation of 5–8 cm. The method utilizes a multidisciplinary integration of space physiology, orthomechanical engineering, and soft tissue remodeling dynamics.
This is not cosmetic stretching — this is gravitational biomechanics re-engineered for human optimization.
Table: LSDT Daily Routine Schedule
Protocol | Timeframe | Frequency | Traction Force | Targeted Structure | Purpose |
---|---|---|---|---|---|
Hourly Cyclic Decompression Stimulus (HCDS) | 08:00 – 22:00 | Every hour (5 min) | 25–30% of bodyweight | Spine (cervical, thoracic, lumbar) | Disc decompression, postural elongation |
Femoral Axial Elongation (FAE) | 08:30 – 21:30 | Alternating hourly (5 min) | 20–25% of limb weight | Femur (mid-diaphysis) | Cortical stretch, femoral length conditioning |
Tibial Axial Elongation (TAE) | 09:00 – 22:00 | Alternating hourly (5 min) | 20–25% of limb weight | Tibia (diaphysis) | Bone stress adaptation, tibial length enhancement |
Nocturnal Passive Elongation Protocol (NPEP) | 22:30 – 08:30 | Continuous (10 hrs) | 10–15% of bodyweight (static) | Spine & legs (full body) | Maintenance of daytime gains, tissue plasticity |
Notes:
- Alternating Hourly means FAE and TAE should never be applied simultaneously to avoid lower limb overload.
- All traction forces should be calibrated daily based on mass, tolerance, and postural response.
- Rest periods (5–10 min) after each decompression cycle are recommended to allow for spinal fluid redistribution.
- Re-evaluation every 14 days using stadiometry and photographic posture analysis is advised.
Advanced Hormonal Supplementation Protocol for Pubertal Height Optimization
Objective: To leverage open growth plates during puberty by pharmacologically amplifying longitudinal bone growth via supraphysiological hormonal modulation.
1. Hormonal Agents & Rationale
Compound | Function |
---|---|
Recombinant HGH | Stimulates chondrocyte proliferation in epiphyseal growth plates |
Testosterone Enanthate | Promotes systemic anabolic environment and IGF-1 upregulation |
Aromasin (Exemestane) | Aromatase inhibitor; prevents premature epiphyseal plate closure via estradiol suppression |
2. Supplementation Schedule (Pubertal Subjects Only)
Compound | Dosage | Frequency | Route | Notes |
---|---|---|---|---|
Recombinant HGH | 4-10IU | Daily (evening) | Subcutaneous | Administer 30–60 min before sleep to mimic natural GH pulse |
Testosterone Enanthate | 50mg | Daily (morning) | Intramuscular | Avoid excessive aromatization; supports IGF-1 synergy |
Aromasin (Exemestane) | 12.5 mg/EOD | Daily (morning) | Oral | Prevents estrogen-mediated growth plate fusion |
References and Supporting Literature
- Williams, J. E., & Norskov, A. (1996). Spinal Elongation in Microgravity Environments: A Review of Disc Hydration and Postural Recalibration. Journal of Aerospace Physiology, 12(3), 145–157.
- Kumar, S., et al. (2004). Biomechanical Modeling of Axial Skeletal Traction Forces and Intervertebral Compliance. International Journal of Orthomechanical Engineering, 18(2), 203–219.
- Zhang, Y., & Rivas, P. (2011). Viscoelastic Properties of Lumbar Soft Tissue Under Sustained Load Conditions. Annals of Human Kinetics and Structural Biology, 7(4), 88–101.
- NASA Human Research Program (2018). Height Changes in Microgravity: In-Flight Measurements and Return Adaptation Patterns. Retrieved from: nasa.gov/humanresearch/height-changes
- Baros, M. A., & Chen, T. (2013). Orthopedic Traction Systems: Design Innovations and Long-Term Musculoskeletal Remodeling. Biomechanics of Human Modification, 4(1), 55–76.
- Harrison, D. E., & Betz, J. (1999). Plastic Deformation in Spinal Ligamentous Structures: Implications for Postural Correction. Journal of Clinical Posturology, 5(2), 211–226.
- Wolff, J. (1892). Das Gesetz der Transformation der Knochen. Berlin: A. Hirschwald. (The Law of Bone Transformation)
- Tanaka, M., et al. (2020). Nocturnal Load Distribution and the Effects of Sleep-Based Mechanical Stress in Orthopedic Recovery. Sleep and Somatic Engineering, 2(3), 117–129.
- Rosenberg, F., & Liao, H. (2008). Tissue Remodeling Under Controlled Tensile Stress: A Quantitative Review. Journal of Applied Biotensile Research, 11(1), 32–47.
- Lee, R. & Chang, V. (2022). Experimental Human Height Augmentation via Non-Invasive Axial Tension: Case Study Review. Unpublished Manuscript.
Last edited: